Fit-for-Purpose Antibodies
Antibodies have become essential tools for research, diagnostic and therapeutic purposes because of their high specificity, high binding affinity, long half-lives, and low toxicity. Antibodies secreted by a single clone of B lymphocytes are termed monoclonal antibodies and those produced by a mixture of various B lymphocyte clones are termed polyclonal antibodies. Antibodies are invaluable reagents for antigen detection and purification, e.g. immunoblotting, immunoprecipitation, immunohistochemistry, ELISA, and immunoaffinity chromatography.
Antibodies are generated by immunizing host animals with an immunogenic material. Antibodies can be produced against a wide range of different immunogens. These immunogens can be full-length proteins, protein fragments, peptides, whole organisms (bacteria), or cells. Thus, given enough time, any foreign substance will be recognized by the immune system and induce specific antibody production. However, this specific immune response is highly variable and depends much in part on the size, structure, and composition of antigens.
Proteins or glycoproteins are generally considered the most suitable antigens due to their structural complexity and size and are thus strongly immunogenic. Lipids usually are not immunogenic but can be made immunogenic by conjugation to a carrier protein. Similarly, nucleic acids are poor immunogens but can become immunogenic when coupled to a carrier protein. Immune responses against small substances and peptides (haptens), can be generated by chemically coupling them to a larger carrier protein, such as bovine serum albumin, keyhole limpet hemocyanin (KLH), or other synthetic matrices.
Successful production of antibodies depends upon careful planning and implementation of critical steps that may influence the outcome of the effective antibody responses. The several important steps and considerations involved in the production of antibodies include the following:
1 Selection of a Right Immunogen
A key action in developing an antibody that works in the intended assay is the selection of an appropriate immunogen. The three characteristics that a substance must have to be immunogenic are foreignness, high molecular weight, and chemical complexity. The most natural immunogens are macromolecules composed of protein, carbohydrate, or a combination of the two. Peptides may have the complexity essential to be antigenic, but their small size typically renders them ineffective as immunogens on their own. Peptides are often covalently coupled to carrier proteins to ensure that they induce an immune response.
If adequate supply of the full-length protein is available, immunization with the full-length protein in the form of native, recombinant, fusion, gel band, etc. may be a convenient and cost-effective option. One characteristic of large antigen molecules is that they induce the activation of numerous antibody-producing B cell clones. This polyclonal mixture of resulting antibodies may then recognize multiple epitopes on the antigen. As a result, there is a very high probability that antibodies against at least one of these epitopes will bind with the native protein in the target assay.
The disadvantage with this approach is that since antibodies are being generated against multiple epitopes, there is a higher chance that antibodies against some of these epitopes could recognize other proteins that contain homologous epitopes. As a result, non-specific cross-reactivity against homologous epitopes might be problematic when assaying with antibodies developed against the full-length protein. Additionally, recombinant expression of a protein is sometimes costly and the expressed protein may not completely resemble all the characteristics of a native protein.
The second strategy is to immunize with a peptide sequence corresponding to a specific region of the full-length protein. With this approach, synthetic peptides are generated which mimic selected regions of the protein of known amino acid sequence. Peptides are frequently favored since they are easy to synthesize in high purity and are easy to work with. Using this approach, antibodies can be raised against selected regions, such as highly conserved regions, active sites, extra- or intra-cellular domains or regions of post-translational or chemical modifications.
The disadvantage of using a peptide sequence is that it is identical to a very specific region of the native protein and this region may not be accessible in the protein’s native conformation in a particular assay.
Some general key considerations in designing de novo or native-based peptides which will increase the probability of generating a successful working antibody are as follows:
- Choose areas of structural stability and chemical complexity within the molecule.
- Select sequences lacking extensive repeat units.
- Avoid complex and inaccessible regions such as alpha helices and beta sheets. Aim for accessible regions of native protein that are surface oriented, hydrophilic, and flexible.
- N and C-terminus are often exposed parts of the protein with a high degree of flexibility and are a good choice for generating anti-peptide antibodies directed against the intact protein.
- Avoid domains that are present in other proteins as these may increase cross reactivity.
- Peptide length in general should be in the range of 10-20 amino acid residues. Peptide sequences of this length minimize synthesis problems, are reasonably soluble in aqueous solution, and may have some degree of secondary structure.
- Determine the regions that should be avoided or targeted, for example, post-translational modification sites such as phosphorylation, glycosylation, ubiquitination, methylation, acetylation, proteolysis.
- Examine the protein sequences for which the resulting antibody should or should not cross-react with.
- Avoid N-terminal glutamine or asparagine and C-terminal proline or glycine in a peptide.
- Avoid internal cysteine (which can be replaced with serine) as cysteine is susceptible to rapid oxidation which can negatively influence the cleavage of protecting groups during synthesis and the subsequent peptide purification.
2 Selection of Right Detection Method
Antibodies for research applications are most commonly used to identify and localize intracellular and extracellular proteins. Antibodies are used in flow cytometry to differentiate cell types by the proteins they express. They are also used in Western blot analysis to detect proteins separated by electrophoresis, in immunoprecipitation to separate proteins and any bound molecules (co-immunoprecipitation) in a cell lysate, and in immunohistochemistry or immunofluorescence to study protein expression in tissue sections or to localize proteins within cells. Proteins can also be detected and quantified with antibodies using ELISA.
It is important to determine the best application for the research need as not all antibodies will work with every application. Qualitative and quantitative applications have vastly different antibody requirements and a selection of an appropriate antibody that works in the intended assay is a key requirement.
For efficient interaction between the target antigen and the antibody, the epitope must be readily accessible for binding. If the target molecule is denatured, e.g., through fixation, reduction, pH changes, or during preparation, the epitope may be changed and this may affect its capability to interact with an antibody. Some antibodies, for example, are ineffective in Western blotting but are appropriate for immunohistochemical applications, because, in immunohistochemistry, a complex antigenic site might be maintained in the tissue, whereas in the Western blotting, the proteins are subjected to denaturing conditions which changes the protein conformation sufficiently to destroy the antigenic site, and hence eliminates antibody binding. Thus the antibodies produced against native proteins are likely to react best with native proteins (e.g. immunoprecipitation or flow cytometry) and antibodies produced against denatured proteins react with proteins subjected to denaturing conditions (e.g. Western blotting).
Optimally, an antibody that identifies a linear epitope on the surface of a normally folded protein will work well in both non-denaturing and denaturing procedures. Thus, the epitope may be present in the antigen’s native, cellular environment, or it may be exposed only when denatured. In their normal form, antigens may be cytoplasmic, membrane-bound, or secreted. The number, location, and size of the epitopes depend on how much of the antigen is presented during the antibody development process.
3 Selection of Right Clonality
Antibodies are produced and purified in two basic forms for use as reagents in immunochemical techniques; polyclonal and monoclonal. Normally, the immunological response to an antigen is heterogeneous, resulting in many different cell lines of B lymphocytes producing antibodies to the same antigen. As a result of this heterogeneous response, several antigen-specific antibody clones, potentially of several different immunoglobulin classes and subclasses are produced. An antibody purified from this heterogeneous collection of antigen-binding immunoglobulins is called a polyclonal antibody.
An individual B lymphocyte produces and secretes a homogenous population of antibodies called monoclonal antibodies. All antibodies secreted by a B cell clone are identical, providing a source of homogenous antibody with a single defined specificity. Monoclonal antibodies can be raised by fusion of B lymphocytes with immortal cell cultures to produce hybridomas. Hybridomas produce many copies of the same antibody. This remarkable phenomenon has been instrumental in the development of antibodies for diagnostic applications since monoclonal antibodies react with one epitope on the antigen.
Additionally, synthetic antibodies called recombinant antibodies can be created using synthetic genes expressed in an in vitro mammalian cell line. Recombinant antibodies are monoclonal antibodies that do not need hybridomas and animals in the production process. The technology involves isolating antibody genes from source cells, amplifying and cloning the genes into an appropriate phage vector, introducing the resulting vector into expression hosts such as bacteria, yeast, or mammalian cell lines, and attaining expression of sufficient amounts of functional antibody. Recombinant antibodies can be used in all applications where classical monoclonal antibodies are used.
Properties of Polyclonal, Monoclonal, and Recombinant Antibodies
Polyclonal Antibodies
- Polyclonal antibodies have a broader specificity and often recognize multiple epitopes, making them less sensitive to minor antigen changes (for example, polymorphism, heterogeneity of glycosylation or slight denaturation). Hence, they can identify proteins of high homology or from different species.
- Polyclonal antibodies are frequently the preferred choice for detecting denatured proteins.
- Polyclonal antibodies are relatively easy to generate, less complex, and are more cost-effective.
- Polyclonal antibodies may be generated in a variety of species, including rabbit, goat, sheep, donkey, chicken, and others, giving the users many options in experimental design.
- Polyclonal antibodies target multiple epitopes on the same protein and thus usually provide more robust detection.
- Polyclonal antibodies are sometimes used when the nature of the antigen in an untested species is not known.
- Polyclonal antibodies can amplify signals from a target protein with low expression levels, as the target protein will bind more than one antibody molecule.
- Polyclonal antibodies are compatible with a wider range of applications.
Monoclonal Antibodies
- Monoclonal antibodies are highly specific and detect only one epitope on the antigen.
- Due to their specificity, monoclonal antibodies are excellent as the primary antibody in an application, or for detection of antigens in tissue, and often minimize background signal and eliminate cross-reactivity.
- The highly specific nature of the monoclonal antibody permits the development of assays where two very closely related antigens can be distinguished from each other.
- Homogeneity of monoclonal antibodies is very high and they provide consistent, reproducible results if experimental conditions are kept constant.
- Hybridoma cells can serve as an infinite source of the monoclonal antibody.
- Monoclonal antibodies are essential for research, diagnostic, and therapeutic applications.
Recombinant Antibodies
- Recombinant antibodies suitable for research purposes can be produced in significantly less time than hybridoma-based methods.
- Recombinant antibodies are developed from a unique set of genes, making them more reliable, and providing controlled and reproducible results.
- Recombinant antibodies can be readily optimized, as their nucleic acid sequences are defined and easily available. A high degree of control is possible, making selection of recombinant antibodies that bind in a particular pH, salinity, or in other specific buffer conditions precise and practical.
- Mass production of recombinant antibodies can be achieved at a shorter timeframe and does not require the use of animals - thus overcomes ethical concerns over animal distress, discomfort, and pain.
- A required recombinant antibody fragment can readily be converted into a different species, isotype, or subtype by adding the appropriate constant domain. This makes it easier to switch antibodies into a more desirable format.
4 Selection of the Right Host
A number of animals may be applied as hosts for production of antibodies, including rabbits, chickens, goats, sheep, cows, mice, guinea pigs, and rats. Selection of the most suitable animal depends on factors such as presence of a homologous protein in the immunized species, the amount of antibody required, the amount of protein available for immunization and the time period required to obtain an antibody response.
The most cost-effective hosts are chickens and rabbits. While chickens are now the second most used host, rabbits are traditionally the most common host animals for polyclonal antibody production. Rabbits are used in 95% of the cases, since they have the capability to respond to broad classes of antigens and provide good yields in a short period of time. Chickens are considered as the host if it is essential for the phylogenetic relationship between the antigen donor and the antibody producer to be distant. Chickens transfer high quantities of immunoglobulins, (IgY), into the egg yolk, which eliminates the need for invasive bleeding procedures.
Goat/sheep is the host of choice for producing polyclonal antibodies in larger quantities. Goat/sheep give strong immune responses against most antigens. The goat produces about 7-8 times the amount of serum compared to the rabbit. Furthermore, the goat serum contains about 20 mg/ml of total IgG, which is about 2-3 times more than rabbit serum. This makes the goat the more cost-effective choice of the two when greater amounts of antibodies are required.
Mice/Rats are used as hosts for screening potential antigens prior to immunization into a larger host. They only provide small amounts of serum.
The most common host for monoclonal antibody production is the mouse. Balb-c mice are usually an obvious choice; they are an inbred strain preferably suited to monoclonal antibody work.
5 Selection of Post-Translational Modification Antibodies
Most proteins are frequently subjected to some form of modification following translation. These post-translational modifications (PTMs) result in mass changes that are detected during analysis. Post-translational modification of proteins increases their functional diversity by the covalent addition of functional groups or proteins, proteolytic cleavage of regulatory subunits, or degradation of entire proteins. These modifications include phosphorylation, glycosylation, ubiquitination, nitrosylation, methylation, acetylation, lipidation, proteolysis, and influence almost all aspects of normal cell biology and pathogenesis. Therefore, the analysis of protein post-translational modifications is particularly important in the study of cell biology and disease treatment and prevention such as heart disease, cancer, and diabetes.
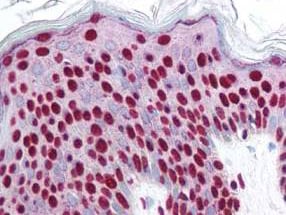
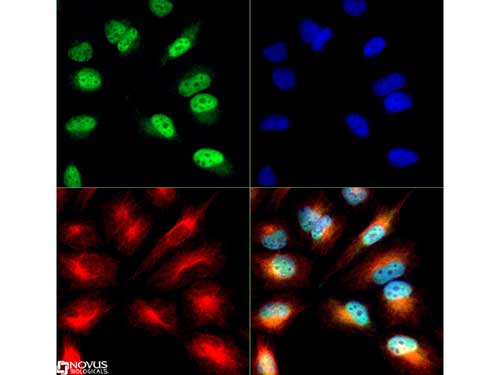
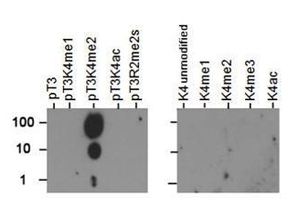
The most widespread and useful tool for tracking post-translational changes is the modification-specific antibody. Such modified-protein-specific antibodies can be used in a wide range of biochemical assays, such as Western blotting, chromatin immunoprecipitation, and flow cytometry.
Antibodies against PTMs are generated using synthetic peptides designed against a short, specific region of the protein, largely eliminating the issue of specificity compared to antibodies generated using large constructs as immunogens. However, it is critical that the antibody is tested against established positive and negative controls to ensure specificity for the modification.
PTM specific antibodies may be either monoclonal or polyclonal. The latter are easier and faster to develop, but both require significant validation. PTM antibodies are purified to remove antibodies that react to the non-modified protein by subtraction against the unmodified peptide, followed by affinity enrichment for antibodies that react to the modified protein. The antibodies bound to the modified peptide are then eluted and tested under various experimental conditions.
6 Antibody Fragments and Subclasses
A range of applications where Fc mediated effects are not required and are even undesirable, the antibody fragments that lack the FC domain are used. The antibody fragments of primary interest are antigen-binding fragments such as Fab' and F(ab')2. Antibody fragments are smaller than whole IgG molecules and are often used to block a signaling molecule or receptor. The smaller size of these antibody fragments offers better tissue delivery resulting in improved staining such as immunohistochemistry and immunocytochemistry. Fab and F(ab')2 fragment antibodies eliminate non-specific binding between Fc portions of antibodies and Fc receptors on cells such as macrophages, dendritic cells, neutrophils, NK cells, and B cells. Furthermore, antibody fragments are frequently used as the starting point for drug molecules because of their lower immunogenicity than intact antibodies.
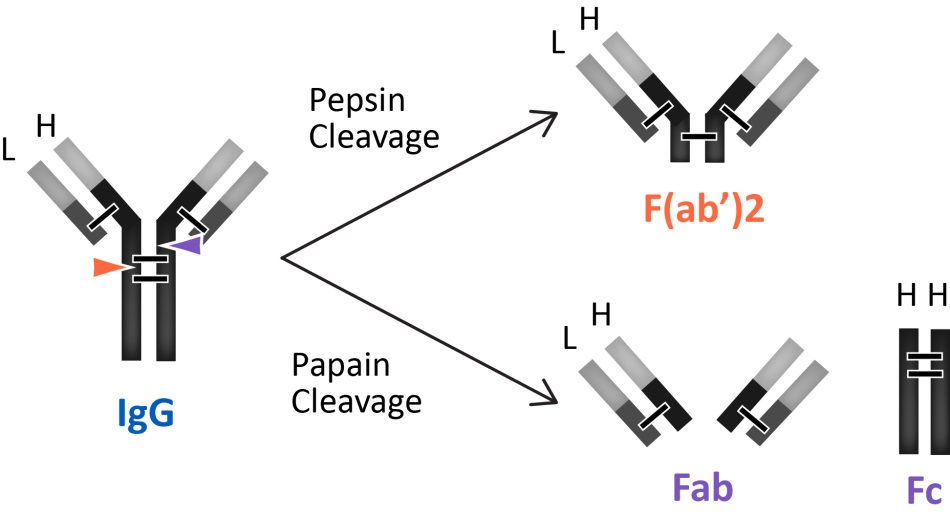
The antibodies produced by plasma cells are classified by their isotypes that differ in function and antigen responses. Five major antibody isotypes have been identified; IgA, IgD, IgE, IgG, and IgM. The antibody isotypes IgG and IgA are further divided into subclasses (e.g. human IgG1, IgG2, IgG3, IgG4, IgA1 and IgA2) based on additional differences in their amino acid heavy chain sequences. Determining the class and subclass identity of an antibody is important for selecting the method to purify and use these antibodies in immunoassays. For example, if an antibody is determined to be IgM, it cannot be purified effectively with Protein A or G, and it will most likely need fragmentation for use in immunohistochemical procedures.
Antibody isotyping is a critical and valuable characteristic of hybridoma development. Screening is one of the most important stages during hybridoma development to ensure that the most productive and positive clones are selected for further evaluation. Antibody isotype screening at the various development stages of fusion, cloning and subcloning by ELISA, and flow cytometry assays, provides an accurate and specific identity of heavy and light chains produced by hybridomas. Several individual hybridoma samples can be tested by ELISA for anti- IgG1, IgG2a, IgG2b, IgG2c or IgG3, IgA, and IgM as well as kappa and lambda light chains. If a monoclonal antibody is determined to be IgG1 composed of kappa light chains, there is an option to use immobilized Protein L to purify it from culture supernatant without contamination of bovine immunoglobulins from the serum supplement.
7 Selection of Right Reporting System
All antibodies require systems to detect the binding of antigens. The method of detection can be ‘direct” if the label is conjugated to the primary antibody or ‘indirect’ if the label is attached to another molecule, called a ‘secondary reagent or antibody’, and may generate a fluorescent or chromogenic signal. It is important to select a secondary antibody that has specificity for the antibody species and isotype of the primary antibody and is conjugated to a suitable detectable tag or label for detection. Detection tags or labels that may be conjugated to purified antibodies include enzymes, fluorophores, or haptens, such as horseradish peroxidase (HRP), alkaline phosphatase (AP), rhodamine, fluorescein isothiocyanate (FITC), DyLight™, AlexaFluor™, Atto dyes or biotin. Labeling strategies result in the covalent attachment of molecular labels to the target protein in order to facilitate the detection of a labeled protein and its binding partners. While multiple types of labels are available, their diverse uses are preferable for specific applications. Therefore, the type of label and the labeling strategy used must be considered carefully and tailored for each application. Conjugated antibodies are used to detect and quantify antigens using an appropriate detection technique such as flow cytometry, ELISA, Western blotting, immunofluorescence and immunohistochemistry.
The choice of whether to use direct or indirect detection is often dictated by the level of antigen expression. The detection of a highly expressed antigen might be possible using a primary antibody directly conjugated to a label. The direct labeling approach is simple and avoids the problems of non-specific binding with labeled secondary antibodies. Additional advantages of using conjugated primary antibodies are the ability to multiplex with antibodies from same species. Indirect detection methods generally have a higher level of sensitivity and generate a more intense signal. The signal is amplified because several secondary antibodies carrying multiple labels bind to primary antibody, resulting in signal amplification. Furthermore, labeled secondary antibodies are readily available.
8 Setting Limits of Specificity and Sensitivity
There is a growing need for antibodies that are both highly specific and highly sensitive. Specificity refers to the properties of an antibody to bind to one or more antigens (a qualitative measurement) and sensitivity refers to how much antibody is needed to elicit a reaction (a quantitative measurement). However, when choosing antibodies, there is usually a trade-off between sensitivity and specificity. Polyclonal antibodies can offer great sensitivity by virtue of being able to recognize multiple epitopes on a specified antigen target, but this advantage also presents potential drawbacks such as increased nonspecific binding. Monoclonal antibodies, on the other hand, provide the maximum specificity because they only recognize a single epitope, but this restricted targeting results in fewer binding sites for the antibody on an antigen, which translates to reduced overall sensitivity. Validating each antibody using rigorous standards including multiple experimental controls, and, when available, multiple cell types in the recommended applications can verify the sensitivity, specificity, and reproducibility of antibodies.
9 Considering Antibody Lifespan
The amount of antibody needed and the characteristics of the antibodies being made depend on the intended use of the resulting antibody. If a large volume diagnostic product is the objective, or if extensive research utilizing resulting anti-serum for a long term is required, immunizing large animals (i.e. sheep, goat, cow) is a viable approach since these can provide large volumes of antiserum from a single bleeding. On the other hand, if the antiserum is to be used for the analysis of a dozen Western blots, immunizing small animals (i.e. guinea pig, rabbit) is a feasible approach. For most routine work where small volumes of antiserum are required, e.g. <100 mL, the rabbit is the most common species for polyclonal production, while goats and sheep are the species of choice for large-scale production of antiserum. Rabbits typically yield 25 mL/bleed or 50-70 mL/month of antiserum and the expected yield of antiserum from goat is 800 mL/bleed or 2-2.5 L/month. If the antigen of interest is a protein that is conserved in mammals, and a host with a larger phylogenetic distance is required, then chicken is the host of choice. The chicken is more cost-effective and will yield in the order of 400 mg total IgY.
10 Benefits of Antibody Pools
Antibodies that are in high demand often need to be re-manufactured, beginning with the immunization of a host animal. Consequently, the specificity and affinity of these antibodies can vary from batch to batch. Since the specificity of the polyclonal population may drift over time during immunization, this drawback can be addressed by immunizing multiple animals at the same time followed by screening and pooling of antiserum prior to preparation or purification. To ensure consistency, the performance of the final antibody can be compared to previous batches.
In a multiple cohort study, the antiserum may be pooled based on different collection dates from the same host, or the same collection date from multiple hosts. To avoid increased background, antibodies from the same host should be pooled and tested for consistency.